Review Study on Piezoelectric Energy Harvesters and Their Applications
N. K. Dixit1 , K. J. Rangra2 , Y. C Sharma3
Section:Review Paper, Product Type: Journal Paper
Volume-6 ,
Issue-9 , Page no. 607-616, Sep-2018
CrossRef-DOI: https://doi.org/10.26438/ijcse/v6i9.607616
Online published on Sep 30, 2018
Copyright © N. K. Dixit, K. J. Rangra, Y. C Sharma . This is an open access article distributed under the Creative Commons Attribution License, which permits unrestricted use, distribution, and reproduction in any medium, provided the original work is properly cited.
View this paper at Google Scholar | DPI Digital Library
How to Cite this Paper
- IEEE Citation
- MLA Citation
- APA Citation
- BibTex Citation
- RIS Citation
IEEE Style Citation: N. K. Dixit, K. J. Rangra, Y. C Sharma, “Review Study on Piezoelectric Energy Harvesters and Their Applications,” International Journal of Computer Sciences and Engineering, Vol.6, Issue.9, pp.607-616, 2018.
MLA Style Citation: N. K. Dixit, K. J. Rangra, Y. C Sharma "Review Study on Piezoelectric Energy Harvesters and Their Applications." International Journal of Computer Sciences and Engineering 6.9 (2018): 607-616.
APA Style Citation: N. K. Dixit, K. J. Rangra, Y. C Sharma, (2018). Review Study on Piezoelectric Energy Harvesters and Their Applications. International Journal of Computer Sciences and Engineering, 6(9), 607-616.
BibTex Style Citation:
@article{Dixit_2018,
author = {N. K. Dixit, K. J. Rangra, Y. C Sharma},
title = {Review Study on Piezoelectric Energy Harvesters and Their Applications},
journal = {International Journal of Computer Sciences and Engineering},
issue_date = {9 2018},
volume = {6},
Issue = {9},
month = {9},
year = {2018},
issn = {2347-2693},
pages = {607-616},
url = {https://www.ijcseonline.org/full_paper_view.php?paper_id=2916},
doi = {https://doi.org/10.26438/ijcse/v6i9.607616}
publisher = {IJCSE, Indore, INDIA},
}
RIS Style Citation:
TY - JOUR
DO = {https://doi.org/10.26438/ijcse/v6i9.607616}
UR - https://www.ijcseonline.org/full_paper_view.php?paper_id=2916
TI - Review Study on Piezoelectric Energy Harvesters and Their Applications
T2 - International Journal of Computer Sciences and Engineering
AU - N. K. Dixit, K. J. Rangra, Y. C Sharma
PY - 2018
DA - 2018/09/30
PB - IJCSE, Indore, INDIA
SP - 607-616
IS - 9
VL - 6
SN - 2347-2693
ER -
![]() |
![]() |
![]() |
460 | 298 downloads | 205 downloads |
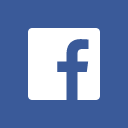
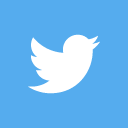
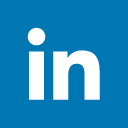
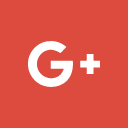
Abstract
To minimize the requirement of external power source and maintenance for electric devices such as wireless sensor networks, the energy harvesting technique based on vibrations has been a dynamic field of studying interest over past years. This presents a challenge for the researchers to optimize the energy output of piezoelectric energy harvesters, due to the relatively high elastic moduli of piezoelectric materials used to date. This paper reviews the current state of research on piezoelectric energy harvesting devices for low frequency (0–100Hz) applications. Various key aspects that contribute to the overall performance of a piezoelectric energy harvester are discussed.
Key-Words / Index Term
Piezoelectric, power sources, optimize energy harvester, Wireless sensor networks
References
[1]. S. Roundy and P. K. Wright, Smart Mater. Struct. 13(5), 1131–1142 (2004).
[2]. S. R. Anton and H. A. Sodano, Smart Mater. Struct. 16(3), R1–R21 (2007)
[3]. Choi WJ, Jeon Y, Jeong JH, Sood R, Kim SG. Energy harvesting MEMS device based on thin film piezoelectric cantilevers. J Electroceram 2006;17(2–4):543–8.
[4]. Shen D, Park J-H, Noh JH, Choe S-Y, Kim S-H, Wikle Iii HC, et al. Micromachined PZT cantilever based on SOI structure for low-frequency vibration energy harvesting. Sens Actuators A: Phys 2009;154(1):103–8.
[5]. Dongna S, Jung-Hyun P, Jyoti A, Song-Yul C, Howard, III CW, Dong-Joo K. The design, fabrication, and evaluation of a MEMS PZT cantilever with an integrated Si proof mass for vibration energy harvesting. J Micromech Microeng 2008;18(5):055017.
[6]. Roundy S, Wright PK, Rabaey J. A study of low level vibrations as a power source for wireless sensor nodes. Comput Commun 2003; 26(11):1131–44.
[7]. Eli SL, Paul KW. Resonance tuning of piezoelectric vibration energy scavenging generators using compressive axial preload. Smart Mater Struct 2006; 15(5):1413.
[8]. DuToit NE, Wardle BL. Experimental verification of models for microfabricated piezoelectric vibration energy harvesters. AIAA J 2007; 45(5):1126–37.
[9]. Dutoit NE, Wardle BL, Kim S-G. Design considerations for mems-scale piezoelectric mechanical vibration energy harvesters. Integr Ferroelectr 2005; 71(1):121–60.
[10]. Erturk A. Electromechanical modeling of piezoelectric energy harvesters. Blacksburg: Virginia Polytechnic Institute and State University; 2009.
[11]. Erturk A, Inman DJ. A distributed parameter electromechanical model for cantilevered piezoelectric energy harvesters. J Vib Acoust 2008; 130(4), [41002].
[12]. Erturk A, Inman DJ. On the mechanical modeling of cantilevered piezoelectric vibration energy harvesters. J Intell Mater Syst Struct 2008;19:15.
[13]. Erturk A, Inman DJ. An experimentally validated bimorph cantilever model for piezoelectric energy harvesting from base excitations. Smart Mater Struct 2009;18(2):025009.
[14]. Erturk A, Inman DJ. Issues in mathematical modeling of piezoelectric energy harvesters. Smart Mater Struct 2008;17(6):065016.
[15]. Roundy S, Leland ES, Baker J, Carleton E, Reilly E, Lai E, et al. Improving power output for vibration-based energy scavengers. Pervas Comput IEEE 2005;4(1):28–36.
[16]. Elfrink R, Kamel TM, Goedbloed M, Matova S, Hohlfeld D, Andel Yv, et al. Vibration energy harvesting with aluminum nitride-based piezoelectric devices. J Micromech Microeng 2009;19 (9):094005.
[17]. Chen Z, Yang Y, Lu Z, Luo Y. Broadband characteristics of vibration energy harvesting using one-dimensional phononic piezoelectric cantilever beams. Physica B: Condens Matter 2013;410:5–12.
[18]. Wei W, Tongqing Y, Xurui C, Xi Y, Qifa Z. Vibration energy harvesting using piezoelectric circular diaphragm array. In: Applications of Ferroelectrics (ISAF/ PFM), 2011 international symposium on and 2011 international symposium on piezoresponse force microscopy and nanoscale phenomena in polar materials. 2011.
[19]. Ericka M, Vasic D, Costa F, Poulin G, Tliba S. Energy harvesting from vibration using a piezoelectric membrane. In: J de Phys IV (Proceedings). EDP Sciences. 2005.
[20]. Minazara E, Vasic D, Costa F, Poulin G. piezoelectric diaphragm for vibration energy harvesting. Ultrasonics 2006; 44(Supplement):Se699–Se703.
[21]. Clark W, Mo C. Piezoelectric energy harvesting for bio-MEMS applications. In: Priya S, Inman D, editors. Energy harvesting technologies. US: Springer; 2009. p. 405–30.
[22]. Sodano HA, Park G, Inman DJ. An investigation into the performance of microfiber composites for sensing and structural vibration applications. Mech Syst Signal Process 2004;18(3):683–97.
[23]. Rezaeisaray M, Gowini ME, Sameoto D, Raboud D, Moussa W. Low-frequency piezoelectric energy harvesting at multi vibration mode shapes. Sens Actuators A: Phys 2015;228:104–11.
[24]. Kymissis J, Kendall C, Paradiso J, Gershenfeld N. Parasitic power harvesting in shoes. In: Wearable computers, 1998. Digest of papers. Second international symposium on. 1998.
[25]. Shenck NS, Paradiso JA. Energy scavenging with shoe-mounted piezoelectrics. Micro IEEE 2001;21(3):30–42.
[26]. 102. Xu, C.D., Ren, B., Di, W.N., Liang, Z., Jiao, J., Li, L.Y., Li, L., Zhao, X.Y., Luo, H.S., and Wang, D. (2012). Cantilever driving low-frequency piezoelectric energy harvester using single crystal material 0.71 Pb(Mg1/3Nb2/3)O3-0.29PbTiO3. Appl. Phys. Lett. 101, 033502.
[27]. Zou, H.-X., Zhang, W.-M., Li, W.-B., Hu, K.-M., Wei, K.-X., Peng, Z.-K., and Meng, G. (2017). A broadband compressive-mode vibration energy harvester enhanced by magnetic force intervention approach. Appl. Phys. Lett. 110, 163904.
[28]. Wu, J., Chen, X., Chu, Z., Shi, W., Yu, Y., and Dong, S. (2016). A barbell-shaped high-temperature piezoelectric vibration energy harvester based on BiScO3-PbTiO3 ceramic. Appl. Phys. Lett. 109, 173901.
[29]. Yang, Z., and Zu, J. (2014). High-efficiency compressive-mode energy harvester enhanced by a multi-stage force amplification mechanism. Energy. Convers. Manag. 88, 829–833.
[30]. Yang, Z., Zhu, Y., and Zu, J. (2015). Theoretical and experimental investigation of a nonlinear compressive-mode energy harvester with a high power output under weak excitations. Smart Mater. Struct. 24, 025028.
[31]. Wang, X., Shi, Z., Wang, J., and Xiang, H. (2016). A stack-based flex-compressive piezoelectric energy harvesting cell for large quasi-static loads. Smart Mater. Struct. 25, 055005.
[32]. Xu, T.-B., Jiang, X., and Su, J. (2011). A piezoelectric multilayer-stacked hybrid actuation/transduction system. Appl. Phys. Lett. 98, 243503.
[33]. 189. Zhao, J., and You, Z. (2014). A shoe embedded piezoelectric energy harvester for wearable sensors. Sensors 14, 12497–12510.
[34]. Jung, W.-S., Lee, M.-J., Kang, M.-G., Moon, H.G., Yoon, S.-J., Baek, S.-H., and Kang, C.-Y. (2015). Powerful curved piezoelectric generator for wearable applications. Nano Energy 13, 174–181.
[35]. Daniels, A., Zhu, M., and Tiwari, A. (2013). Design, analysis, and testing of a piezoelectric flex transducer for harvesting bio-kinetic energy. J. Phys. Conf. Ser. 476, 012047.
[36]. Xie, L., and Cai, M. (2014). Increased piezoelectric energy harvesting from human footstep motion by using an amplification mechanism. Appl. Phys. Lett. 105, 143901.
[37]. Ylli, K., Hoffmann, D., Willmann, A., Becker, P., Folkmer, B., and Manoli, Y. (2015). Energy harvesting from human motion: exploiting swing and shock excitations. Smart Mater. Struct. 24, 025029.
[38]. Ansari, M., and Karami, M.A. (2017). Experimental investigation of fan-folded piezoelectric energy harvesters for powering pacemakers. Smart Mater. Struct. 26, 065001.
[39]. Dagdeviren, C., Yang, B.D., Su, Y., Tran, P.L., Joe, P., Anderson, E., Xia, J., Doraiswamy, V., Dehdashti, B., Feng, X., et al. (2014). Conformal piezoelectric energy harvesting and storage from motions of the heart, lung, and diaphragm. Proc. Natl. Acad. Sci. USA 111, 1927–1932.
[40]. Bedell, S.W., Fogel, K., Lauro, P., Shahrjerdi, D., Ott, J.A., and Sadana, D. (2013). Layer transfer by controlled spalling. J. Phys. D 46, 152002.
[41]. Potkay, J.A., and Brooks K. An arterial cuff energy scavenger for implanted microsystems. in Bioinformatics and Biomedical Engineering, 2008. ICBBE 2008. The 2nd International Conference. 2008. IEEE.
[42]. Zhang, H., Zhang, X.-S., Cheng, X., Liu, Y., Han, M., Xue, X., Wang, S., Yang, F., Smith, A.S., Zhang, H., and Xu, Z. (2015). A flexible and implantable piezoelectric generator harvesting energy from the pulsation of the ascending aorta: in vitro and in vivo studies. Nano Energy 12, 296–304.
[43]. Bowen, C., and Arafa, M. (2015). Energy harvesting technologies for tire pressure monitoring systems. Adv. Energy Mater. 5, https://doi.org/10.1002/aenm.201401787.
[44]. Kubba, A.E., and Jiang, K. (2014). A comprehensive study on technologies of tyre monitoring systems and possible energy solutions. Sensors (Basel) 14, 10306–10345.
[45]. Mak, K.H., McWilliam, S., and Popov, A.A. (2013). Piezoelectric energy harvesting for tyre pressure measurement applications. J. Automobile Eng. 227, 842–852.
[46]. Van Schaijk, R., Elfrink, R., Oudenhoven, J., Pop, V., Wang, Z., and Renaud, M. (2013). A MEMS vibration energy harvester for automotive applications. Proc. SPIE. https:// doi.org/10.1117/12.2016916.
[47]. Elfrink, R., Matova, S., de Nooijer, C., Jambunathan, M., Goedbloed, M., van de Molengraft, J., Pop, V., Vullers, R.J.M., Renaud, M., and van Schaijk, R. (2011). Shock-induced energy harvesting with a MEMS harvester for automotive applications. In Electron Devices Meeting (IEDM), 2011 IEEE International. IEEE.
[48]. Zheng, Q., Tu, H., Agee, A., and Xu, Y. (2009). Vibration energy harvesting device based on asymmetric air-spaced cantilevers for tire pressure monitoring system. Proceedings of Power MEMS, 403–406.
[49]. Sadeqi, S., Arzanpour, S., and Hajikolaei, K.H. (2015). Broadening the frequency bandwidth of a tire-embedded piezoelectric-based energy harvesting system using coupled linear resonating structure. IEEE/ASME Trans. Mechatronics 20, 2085–2094.
[50]. Zhu, B., Han, J., Zhao, J., and Deng, W. (2017).The practical design of an energy harvester considering wheel rotation for powering intelligent tire systems. J. Electron. Mater. 46, 2483–2493.
[51]. Jousimaa, O.J., Parmar, M., and Lee, D.-W. (2016). Energy harvesting system for intelligent tyre sensors. in Intelligent Vehicles Symposium (IV), 2016 IEEE. IEEE.
[52]. Wu, X., Parmar, M., and Lee, D.-W. (2014). A seesaw-structured energy harvester with superwide bandwidth for TPMS application. IEEE/ASME Trans. Mechatronics 19, 1514–1522.
[53]. Roundy, S., and Tola, J. (2014). Energy harvester for rotating environments using offset pendulum and nonlinear dynamics. Smart Mater. Struct. 23, 105004.
[54]. Erturk, A. (2011). Piezoelectric energy harvesting for civil infrastructure system applications: moving loads and surface strain fluctuations. J. Intell. Mater. Syst. Struct. 22, 1959–1973.
[55]. Zhang, Y., Cai, S.C., and Deng, L. (2014). Piezoelectric-based energy harvesting in bridge systems. J. Intell. Mater. Syst. Struct. 25, 1414–1428.
[56]. Xie, X., Wu, N., Yuen, K.V., and Wang, Q. (2013). Energy harvesting from high-rise buildings by a piezoelectric coupled cantilever with a proof mass. Int. J. Eng. Sci. 72, 98–106.
[57]. Cahill, P., Nuallain, N.A.N., Jackson, N., Mathewson, A., Karoumi, R., and Pakrashi, V. (2014). Energy harvesting from the train-induced response in bridges. J. Bridge Eng. 19, 04014034.
[58]. Karimi, M., Karimi, A., Tikani, R., and ZiaeiRad, S. (2016). Experimental and theoretical investigations on piezoelectric-based energy harvesting from bridge vibrations under traveling vehicles. Int. J. Mech. Sci. 119, 1–11.
[59]. Bhaskaran, P.R., Rathnam, J.D., Koilmani, S., and Subramanian, K. (2017). Multiresonant frequency piezoelectric energy harvesters integrated with high sensitivity piezoelectric accelerometer for bridge health monitoring applications. Smart Mater. Res. 2017, https://doi.org/10.1155/2017/6084309.
[60]. Maruccio, C., Quaranta, G., DeLorenzis, L., and Monti, G. (2016). Energy harvesting from electrospun piezoelectric nanofibers for structural health monitoring of a cable-stayed bridge. Smart Mater. Struct. 25, 085040.
[61]. Peigney, M., and Siegert, D. (2013). Piezoelectric energy harvesting from traffic-induced bridge vibrations. Smart Mater. Struct. 22, 095019.
[62]. Lee, S., Youn, B.D., and Jung, B.C. (2009). Robust segment-type energy harvester and its application to a wireless sensor. Smart Mater. Struct. 18, 095021.
[63]. Rhimi, M., and Lajnef, N. (2012). Tunable energy harvesting from ambient vibrations in civil structures. J. Energy Eng. 138, 185–193.
[64]. Zhang, Y., Cai, C., and Zhang, W. (2014). Experimental study of a multi-impact energy harvester under low-frequency excitations. Smart Mater. Struct. 23, 055002.